Blogs
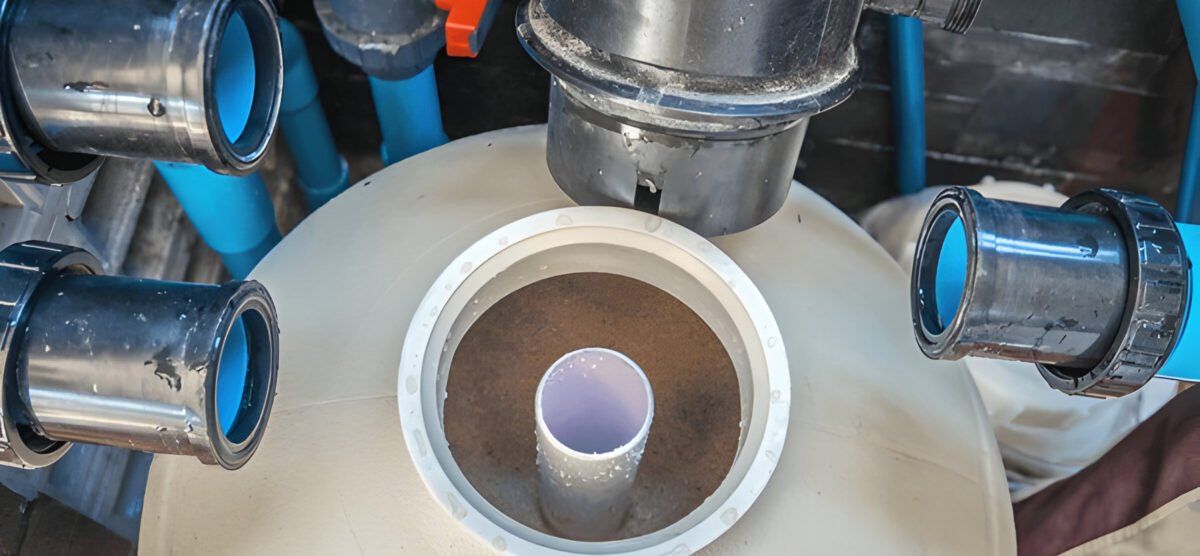
How Does Sputtering Target Work: A Comprehensive Guide
Understanding how sputtering targets work is essential for anyone involved in thin film deposition—a technique widely used across industries like electronics, optics, and renewable energy. These targets are the heart of a process known as sputtering, where atoms are ejected from a source material and deposited as ultra-thin layers onto a surface. This technique is key to creating high-performance coatings, semiconductors, and advanced materials.
In this guide, we’ll break down how sputtering targets function, explain the sputtering process in simple terms, and explore their role in various applications. By the end, you’ll have a clear grasp of how this technology powers modern manufacturing.
What is a Sputtering Target?
A sputtering target is the material from which atoms are ejected during the sputtering process. It serves as the source for creating thin films via physical vapor deposition (PVD). The choice of target material depends on the desired properties of the film—such as electrical conductivity, durability, or optical clarity.
Common Types of Sputtering Targets
- Metal Targets
Metals like gold, silver, copper, aluminum, and titanium are frequently used for their excellent conductivity and versatility. These are essential in electronics where conductive layers are needed. - Ceramic Targets
Ceramics like aluminum oxide, silicon dioxide, and titanium dioxide offer hardness, chemical resistance, and thermal stability. They’re ideal for optical lenses and protective coatings. - Alloy Targets
Alloys combine two or more metals to achieve custom properties. For instance, nickel-chromium alloys provide both conductivity and corrosion resistance—great for demanding environments. - Composite Targets
These mix materials, such as metal-ceramic combinations, to produce thin films with unique traits—like high hardness and thermal resistance combined with electrical performance.
The Sputtering Process, Explained Simply
The sputtering process is a high-tech method used to deposit thin films by physically dislodging atoms from a target. Here’s how it works:
Step-by-Step Overview
- Plasma Generation
Inside a vacuum chamber, an inert gas like argon is introduced. A high voltage ionizes the gas, forming a plasma—a mix of positively charged ions and electrons. - Ion Bombardment
The target (held at a negative voltage) attracts the ions. When these ions collide with the target, they knock atoms off its surface. - Thin Film Deposition
The ejected atoms travel through the vacuum and land on the substrate, forming a thin, uniform film.
Types of Sputtering Techniques
- DC Sputtering: Best for conductive materials like metals, using direct current to generate plasma.
- RF Sputtering: Uses radio frequency power to sputter non-conductive materials, like ceramics, while avoiding charge buildup.
- Magnetron Sputtering: Enhances efficiency using magnets to trap electrons near the target, increasing ionization and improving deposition rates.
Understanding these techniques is key to optimizing how sputtering targets work across different materials and applications.
The Mechanism Behind Sputtering Targets
The basic principle involves momentum transfer: when high-energy ions hit the target, they transfer energy to its atoms, knocking them loose in a process known as sputtering.
Factors Influencing Sputtering Efficiency
- Ion Energy: Higher-energy ions increase the sputtering yield.
- Target Material: Materials with lower atomic bonding energies sputter more easily.
- Angle of Incidence: Ions hitting at an angle can dislodge more atoms than those hitting straight on.
- Plasma Conditions: Pressure, gas type, and magnetic fields all affect how efficiently atoms are ejected.
Carefully controlling these factors ensures consistent, high-quality thin film deposition.
How Sputtering Targets Deposit Thin Films
The functionality of a sputtering target lies in its ability to transfer atoms from solid to surface—accurately and evenly. The environment in the sputtering chamber plays a huge role in the success of this process.
Key Elements of the Thin Film Formation Process
- Controlled Vacuum Environment: Prevents contamination and allows atoms to reach the substrate undisturbed.
- Layer-by-Layer Deposition: Films are built atom by atom, ensuring precision in thickness and composition—especially critical in semiconductors.
Industrial Applications of Sputtering Targets
Sputtering targets are used in a wide range of industries, each with unique requirements for the films they produce:
- Semiconductors: For depositing metal layers on silicon wafers in integrated circuits.
- Optics: To create anti-reflective and protective coatings on lenses and mirrors.
- Solar Panels: For applying thin film materials like cadmium telluride that enhance photovoltaic performance.
These applications highlight the versatility of sputtering targets in producing thin films with exacting standards.
The Role of Sputtering Targets in Semiconductor Manufacturing
In semiconductors, sputtering is indispensable for building the tiny, complex layers of integrated circuits (ICs). Each layer must be deposited with extreme precision.
Types of Layers Created by Sputtering
- Conductive Layers: Metals like copper and tungsten form the electrical pathways.
- Barrier Layers: Materials like titanium nitride prevent metal diffusion between layers.
- Dielectric Layers: Insulators such as silicon dioxide separate conductive elements.
Thanks to sputtering, these layers can be applied with atomic-scale accuracy—enabling faster, smaller, and more powerful electronics.
Latest Advancements in Sputtering Target Technology
As demand for better performance grows, sputtering technology is evolving rapidly.
Recent Innovations
High-Purity Targets: Minimize film defects, especially important for semiconductors and optics.
Advanced Magnetron Designs: Improve deposition rates and film uniformity—crucial for large-scale production.
Reactive Sputtering: Introduces reactive gases like oxygen or nitrogen to form compound films (e.g., oxides, nitrides) with custom properties.
Challenges and Future Trends
Despite progress, challenges remain:
- Material Costs: High-purity targets can be expensive; cost-effective alternatives are being explored.
- Deposition Uniformity: Uniform coverage on large or complex surfaces remains tricky.
- Target Utilization: Improving how fully a target is consumed reduces waste and cost—solutions like rotating targets are helping.
Future advancements will continue pushing the boundaries of what sputtering can achieve, especially in AI chips, flexible electronics, and advanced displays.
Conclusion
Sputtering targets are the foundation of modern thin film deposition. From creating smartphone screens to building solar panels and semiconductors, they play a vital role in many industries. By understanding how sputtering targets work—and how to control the variables involved—you can unlock high-performance coatings and materials with precision and efficiency.
Frequently Asked Questions
Q1. How does a sputtering target work?
A sputtering target releases atoms when struck by high-energy ions. These atoms travel through a vacuum and deposit on a surface, forming a thin film.
Q2. What materials are used in sputtering targets?
Targets can be made from metals, ceramics, alloys, or composites, based on the film properties needed.
Q3. What is the role of sputtering in semiconductor manufacturing?
Sputtering deposits the thin layers required for integrated circuits, including conductors, barriers, and insulators.
Q4. How is magnetron sputtering different?
It uses magnets to boost efficiency, increasing ion density and improving film uniformity and speed.
Q5. What affects sputtering efficiency?
Factors include ion energy, material properties, angle of ion impact, and plasma settings.
Q6. Can non-conductive materials be sputtered?
Yes—RF sputtering is used to safely deposit non-conductive films like ceramics.
Q7. What is reactive sputtering?
It adds reactive gases (e.g., oxygen or nitrogen) into the chamber to create compound films such as oxides and nitrides.
Q8. What are the latest advancements in sputtering technology?
Trends include higher-purity targets, improved magnetron systems, and expanded use of reactive sputtering for complex films.