Blogs
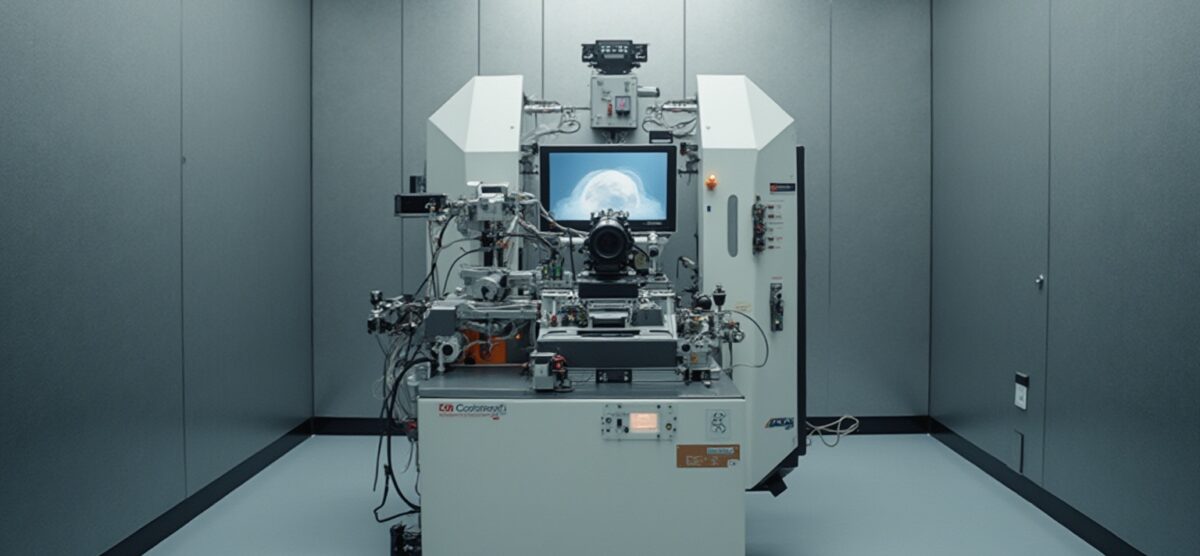
Understanding Magnetometers Shielding in Magnetoencephalography and Magnetocardiography: A Complete Tutorial
Overview:
The article focuses on the importance of magnetometers shielding in magnetoencephalography (MEG) and magnetocardiography (MCG), highlighting their role in accurately measuring weak magnetic fields generated by neural and cardiac activities. It emphasizes that advancements in shielding materials and techniques, such as the use of mu-metal and the design of magnetically shielded rooms, are crucial for enhancing measurement accuracy and ensuring reliable data quality in clinical applications.
Introduction
The intricate world of magnetometers plays a pivotal role in the fields of magnetoencephalography (MEG) and magnetocardiography (MCG), offering profound insights into the magnetic fields generated by brain and heart activity.
As healthcare technology advances, understanding the fundamentals of these instruments becomes crucial for professionals in procurement, who must navigate the complexities of selecting appropriate equipment.
This article delves into the essential components of magnetometers, the significance of magnetic shielding, and the strategic design of magnetically shielded rooms (MSRs). It also highlights best practices for maintenance and evaluation of shielding materials, ensuring that procurement managers are equipped with the knowledge needed to make informed decisions in acquiring cutting-edge technology for medical applications.
With ongoing advancements and research, this exploration provides a comprehensive overview of how to enhance measurement accuracy and reliability in clinical settings.
Fundamentals of Magnetometers in MEG and MCG
Magnetometers shielding in magnetoencephalography and magnetocardiography are advanced devices designed to accurately measure the strength and direction of fields, playing a crucial role in clinical applications. These devices are essential for detecting the subtle fields generated by neuronal and cardiac activities. Specifically, MEG captures the fields resulting from neural currents, allowing for detailed insights into brain function, while MCG focuses on the signals produced by the heart’s electrical activity.
Among the various types of magnetometers used in these contexts, Superconducting Quantum Interference Devices (Squids) and optically pumped magnetometers (OPMs) are the most prevalent. Recent advancements have emphasized the performance of OPM modules, which, despite an increase in noise after MRI operation, have shown strong sensitivity through differential measurements. This research is supported by the NIBIB (USA) grant R01 EB030896, emphasizing the significance of ongoing funding in advancing magnetometer technology.
Notably, Yosuke Ito states, ‘Y.I. and H.U. declare no potential conflict of interest,’ adding an expert perspective to the discussion.
Furthermore, a study titled ‘Field Strength and Current Dipole Distance’ examined the performance of these devices, revealing the OPM’s potential in magnetoencephalography and magnetocardiography, particularly in the context of magnetometers shielding in magnetoencephalography and magnetocardiography, with the ability to measure signals from current dipoles located up to approximately 48 millimeters from the scalp. Such insights are crucial for procurement managers looking to make informed decisions regarding the acquisition of cutting-edge magnetometer technology for medical applications in 2024.
The Role of Magnetic Shielding in Enhancing Measurement Accuracy
Magnetic protection is a critical aspect of achieving controlled environments for sensitive measurements, effectively blocking or attenuating external magnetic fields. Key materials utilized in this process include mu-metal and permalloy, renowned for their high permeability and effectiveness across various frequency ranges. Superconductors also play a crucial role, particularly in applications requiring extreme protection capabilities.
Techniques such as the construction of magnetically shielded rooms (MSRs) are increasingly employed, with the Light Mushroom serving as a prime example. This facility is designed to counteract the Earth’s geomagnetic field, which can interfere with the magnetization of mu-metal over time. By utilizing demagnetization coils, the Light Mushroom maintains a consistent magnetization state, ensuring that any disruptions caused by door openings are mitigated.
Remarkably, after each door opening, the room attains a vertical remnant field component of 4.84 ± 0.39 nT, effectively protecting against the Earth’s field by a factor of approximately 10,000. Allmendinger et al. employed applied shaking with a low frequency of 0.2 Hz and low current of 1 A to the outermost high-permeability layer of the MSR, demonstrating innovative methods in field protection.
Additionally, the detectable current dipole moment was estimated to be about 15 Nam, with measurements anticipated to be feasible up to approximately 48 millimeters from the scalp. Such advancements emphasize the significance of efficient electromagnetic protection, including magnetometers shielding in magnetoencephalography and magnetocardiography, in improving the signal-to-noise ratio of measurements, resulting in enhanced data quality and precision. For uses requiring high-temperature resistance and electrical insulation, products such as Mica Tape provide exceptional thermal and electrical insulation characteristics, rendering them essential in the assembly of coils and other components that need reliable protection in challenging environments.
When acquiring supplies for shielding against magnetism, it is crucial to assess factors such as permeability, thickness, and the specific measurement criteria to guarantee optimal performance in sensitive uses. Furthermore, the design and optimization of magnetic-shielding devices are essential for achieving ultra-low magnetic environments for sensitive measurements. Furthermore, consider product tags for substances such as Beryllium Copper, Kovar, Mu-Metal, and Nickel, which are essential for various applications.
Customer inquiry support is also available to assist in choosing the right resources for your specific needs.
Designing Magnetically Shielded Rooms (MSRs)
Designing an effective magnetically shielded room (MSR) necessitates a strategic approach that includes considerations for magnetometers shielding in magnetoencephalography and magnetocardiography along with several critical factors. The application of high-permeability substances, specifically mu-metal, is primary for magnetometers shielding in magnetoencephalography and magnetocardiography to protect against low-frequency magnetic fields. As pointed out by Prof. Shiqiang Zheng, ‘The selection of materials greatly affects the effectiveness of protection, especially in sensitive measurements.’
The effectiveness of mu-metal has been widely acknowledged in the context of magnetometers shielding in magnetoencephalography and magnetocardiography, as its relative permeability can vary significantly, from 800 to 15,000, affecting the room’s overall protection efficiency. Dimensions and specifications of the MSR should be meticulously tailored to the specific magnetometer employed, ensuring adequate space for personnel and equipment while considering magnetometers shielding in magnetoencephalography and magnetocardiography. Furthermore, the room’s layout must be carefully planned to mitigate electronic noise from surrounding devices, which is vital for maintaining measurement accuracy, particularly concerning magnetometers shielding in magnetoencephalography and magnetocardiography.
Incorporating non-magnetic fixtures and ensuring proper ventilation are additional considerations that contribute to the overall functionality of the MSR. Collaboration with seasoned architects and engineers who specialize in magnetometers shielding in magnetoencephalography and magnetocardiography is essential for the successful implementation of MSRs. Such partnerships not only facilitate compliance with the latest design standards for magnetically shielded rooms but also enhance the likelihood of achieving optimal measurement fidelity through magnetometers shielding in magnetoencephalography and magnetocardiography.
Recent studies, such as those by Wu et al., have emphasized the significance of structural and electromagnetic properties, particularly magnetometers shielding in magnetoencephalography and magnetocardiography, in the design phase, providing a foundation for informed decision-making in the procurement process. Furthermore, the Bertotti Loss Separation Model demonstrates that hysteresis loss is the primary source of noise in ferrite shields at low frequencies, which highlights the importance of selecting appropriate substances in the context of magnetometers shielding in magnetoencephalography and magnetocardiography design. For individuals engaged in procurement, it is vital to obtain from trustworthy suppliers and manufacturers, encompassing choices for Beryllium Copper, Kovar, Mu-Metal, and Nickel, along with various electrical insulation products like crepe paper, films, and high-temperature fabrics.
This guarantees that the substances fulfill the strict criteria essential for effective protection. Engaging with suppliers who specialize in these products will provide procurement managers with a comprehensive understanding of their options, enhancing the overall effectiveness of their sourcing strategies.
Evaluating Shielding Materials and Their Performance
In evaluating protective substances for MEG and MCG applications, it is crucial to consider a variety of factors that influence magnetometers shielding in magnetoencephalography and magnetocardiography performance. Key among these are permeability, saturation magnetization, and thermal stability. High-permeability substances, especially mu-metal, are preferred for their ability to effectively redirect magnetic field lines, thereby improving protection effectiveness.
Beryllium Copper and Kovar also play essential roles in various uses due to their unique characteristics, such as excellent conductivity and mechanical strength. The thickness of the protective substance is equally significant; while thicker options can enhance performance, they may also result in higher costs, requiring a careful balance between effectiveness and budget limitations.
Moreover, Mica Tape products, recognized for their high-temperature resistance and electrical insulation characteristics, can play a vital role in these applications. Their excellent thermal stability and durability make them indispensable in scenarios where both magnetic shielding and thermal management are necessary. A critical statistic to consider is that the sampling frequency should be 3–4 times higher than the highest frequency of interest, which underscores the importance of precision in measurement systems.
Additional considerations include the substance’s resistance to corrosion, fabrication challenges, and compatibility with existing systems. It is advisable to conduct performance tests in controlled environments to validate the selected substances before full-scale procurement. This empirical method ensures that decisions are substantiated by data, thereby mitigating risks associated with the selection of inadequately evaluated resources.
As noted by Wolters et al.,
Individual surface-based volume conductor models can be constructed from the anatomical MRI, for example, by segmenting one or more surfaces associated with a marked change in conductivity.
This emphasizes the significance of precise modeling in enhancing protective performance for effective MEG and MCG uses, especially concerning magnetometers shielding in magnetoencephalography and magnetocardiography. Moreover, case studies, such as the one on anatomical MRI acquisition for MEG studies, illustrate the practical benefits of acquiring individual anatomical MRIs, reinforcing the significance of accurate modeling and its impact on source localization in MEG studies.
Moreover, time-frequency decomposition methods offer important insights into oscillatory activity linked with evoked responses, further highlighting the necessity for strict performance metrics in assessing protective substances. To ensure a comprehensive understanding of the available materials, it is also essential to consider suppliers and manufacturers of electrical insulation and high-temperature materials, which can provide critical support in selecting the right products for specific applications.
Best Practices for Maintenance of Shielding Systems
To ensure the sustained effectiveness of magnetic protection systems, it is imperative to implement a robust schedule of regular inspections and maintenance. This involves comprehensive examinations for physical integrity, including detecting any dents, corrosion, or degradation that could undermine the performance of the protection. As Dan Xian notes, ‘To avoid the potential electromagnetic radiation damage and disruption of the operation of surrounding equipment, a safe distance needs to be kept.’
Therefore, it is equally vital to assess the potential impact of newly introduced equipment, ensuring that it does not interfere with the current protection. For instance, the AMS system for the n2EDM experiment was implemented on an irregular grid with 308 tiles, showcasing the complexity and necessity of maintaining such systems. Organizations should maintain comprehensive records of maintenance schedules, repairs, and inspections; this documentation is invaluable for identifying performance trends over time.
Furthermore, regular training sessions for staff on the importance of protecting maintenance and the correct handling of equipment can significantly mitigate operational risks. By following these best practices, organizations can uphold the integrity of their field protection systems, thereby facilitating precise measurements in magnetoencephalography and magnetocardiography with magnetometers shielding in magnetoencephalography and magnetocardiography. As emphasized in recent research, including the case study titled ‘Future Directions for Magnetic Shielding Research,’ maintaining magnetometers shielding in magnetoencephalography and magnetocardiography effectiveness is not merely a procedural task but a crucial investment in the precision and reliability of experimental outcomes, highlighting the need for comprehensive studies and increased funding for advancements in magnetic shielding technologies.
Conclusion
The exploration of magnetometers, magnetic shielding, and the design of magnetically shielded rooms (MSRs) underscores the integral role these components play in enhancing clinical measurements in magnetoencephalography (MEG) and magnetocardiography (MCG). By understanding the fundamentals of magnetometer technology, including the advantages of Superconducting Quantum Interference Devices (SQUIDs) and optically pumped magnetometers (OPMs), procurement managers can make informed decisions that directly impact patient outcomes and research advancements.
The importance of effective magnetic shielding cannot be overstated, as it significantly improves measurement accuracy by mitigating external magnetic interference. Utilizing high-permeability materials and innovative shielding designs, such as the Light MuRoom, demonstrates how strategic planning can yield superior data quality. Furthermore, the meticulous design of MSRs, incorporating non-magnetic materials and proper ventilation, ensures an optimal environment for sensitive measurements.
Evaluating shielding materials based on their magnetic properties, thermal stability, and performance metrics is essential for achieving the desired efficacy in clinical applications. Regular maintenance and inspections of shielding systems are also vital to preserving their integrity and ensuring reliable results over time. By adhering to best practices in procurement and maintenance, healthcare professionals can enhance the operational efficiency of magnetometer systems, ultimately leading to greater diagnostic precision and improved patient care.
In summary, the knowledge gained from this article equips procurement managers with the strategic insights necessary to navigate the complexities of magnetometer technology and magnetic shielding. As advancements continue to shape the landscape of medical technology, staying informed and proactive in these areas will be crucial for fostering innovation and success in clinical environments.