Blogs
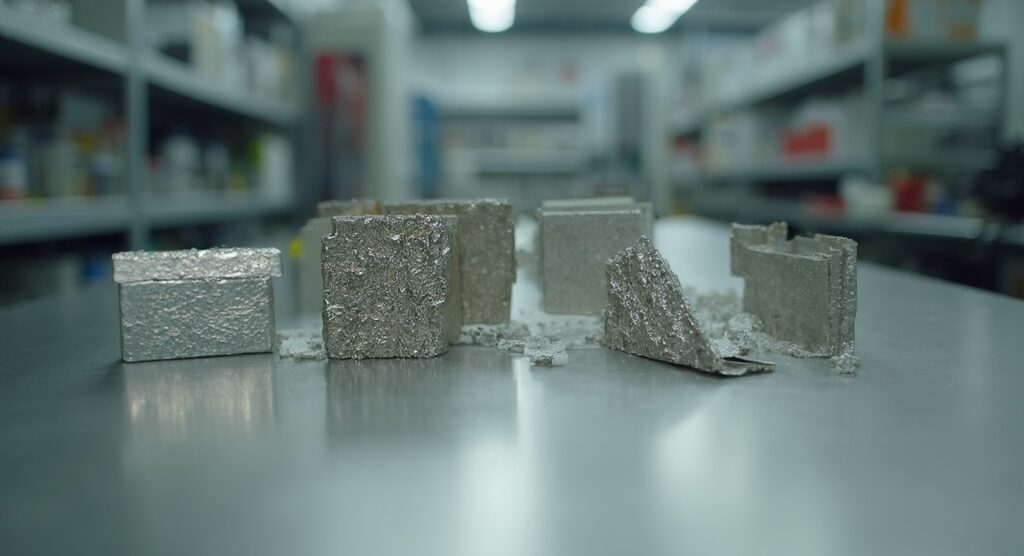
Understanding the Properties and Uses of High Temperature Alloys
Introduction
In the realm of industrial materials, high-temperature alloys stand as a testament to human ingenuity, designed to endure the most extreme thermal environments without compromising their structural integrity. Unlike conventional alloys such as steel and aluminum, which falter under high temperatures or corrosive conditions, advanced high-temperature alloys, particularly multi-principal element alloys (MPEAs), showcase remarkable strength, hardness, and toughness. These alloys are indispensable across various industries, from aerospace to nuclear power, where they must perform reliably under sustained high stress and intense heat.
The article delves into the properties that make these alloys exceptional, examining their mechanical robustness, oxidation resistance, and fatigue resistance. It explores different types of high-temperature alloys—nickel-based, cobalt-based, and iron-based—each with unique benefits tailored to specific applications. Furthermore, the discussion extends to the critical factors influencing the selection of these materials, considering operating temperatures, mechanical stress, and economic aspects.
Highlighting the forefront of material science, the article also addresses the innovative methods driving the development of next-generation alloys, including the use of predictive tools and advanced manufacturing technologies. As industries push the boundaries of what these materials can achieve, the continuous evolution of high-temperature alloys remains a pivotal focus, ensuring their vital role in the most demanding industrial applications.
Properties of High Temperature Alloys
High-temperature materials are carefully crafted to withstand intense thermal conditions while preserving their structural stability. For instance, traditional mixtures such as steel liquefy at approximately 2,500 degrees Fahrenheit, and aluminum deteriorates when in contact with moisture and oxygen. In contrast, advanced high-temperature materials, such as multi-principal element compositions (MPEAs), provide superior performance in extreme conditions, including space and Arctic operations. These MPEAs, composed of several elements in roughly equal proportions, exhibit remarkable strength, hardness, and toughness across a range of temperatures, alongside excellent corrosion resistance and thermal stability.
These metal mixtures excel in applications that demand materials to perform reliably under high stress over extended periods. Their exceptional oxidation resistance enables them to withstand prolonged high-temperature exposure without degradation. Notably, their mechanical properties, such as tensile strength and creep resistance, are crucial for maintaining performance under sustained stress. Furthermore, high-temperature materials exhibit substantial fatigue resistance, rendering them suitable for dynamic loading conditions.
In the aerospace and nuclear power sectors, the creation of new metal mixtures is essential. Researchers have developed tools to forecast how high-entropy materials will act in high-temperature oxidative conditions. For example, materials scientist Arun Devaraj and his team at Pacific Northwest National Laboratory (PNNL) have utilized precision instruments to examine the deterioration of high-entropy mixtures such as the Cantor composition, made up of cobalt, chromium, iron, nickel, and manganese. Their work aims to develop next-generation materials with superior resistance to harsh environments, incorporating rapid sampling, advanced atomic-scale methods, and artificial intelligence to expedite material design.
This innovative method is essential as it allows for the development of mixtures that can withstand harsh conditions, such as those faced in nuclear fusion reactors, hypersonic flights, and high-temperature jet engines. The objective is to create mixtures with outstanding high-temperature characteristics swiftly and effectively, guaranteeing their appropriateness for numerous challenging industrial uses.
Types of High Temperature Alloys
High temperature materials are vital for numerous industrial applications due to their ability to withstand extreme conditions. These metal mixtures can be categorized into nickel-based, cobalt-based, and iron-based types, each offering unique advantages.
Nickel-based materials are celebrated for their exceptional strength and corrosion resistance at elevated temperatures. This makes them indispensable in aerospace and gas turbine applications. The aerospace industry, for instance, is experiencing a surge in demand, driven by new aircraft orders from major airlines like United Airlines and IndiGo. With Boeing and Airbus anticipating more than 2,400 new requests together, the need for nickel composites is driving up prices, despite a decline in raw material expenses. Furthermore, nickel compositions are also essential in other industries such as oil and gas, power generation, and renewable energy. Their use in these industries, ranging from valve assemblies in petrochemical plants to components in nuclear reactors, underscores their versatility.
Cobalt-based mixtures, on the other hand, are recognized for their superior wear resistance. This makes them appropriate for cutting instruments and dental uses. Their robustness ensures longevity and reliability in high-stress environments.
Iron-based mixtures, while generally more cost-effective, are effective in moderately high-temperature environments. They find extensive use in automotive applications where budget constraints often dictate selection of substances. These metal mixtures provide a balance between performance and cost, making them a practical choice for many manufacturers.
In light of rising demands for substances that can withstand extreme conditions, like those found in space and the Arctic, Multi-principal element mixtures (MPEAs) are attracting interest. MPEAs exhibit high strength, hardness, and toughness across a range of temperatures, along with excellent corrosion resistance and thermal stability. This makes them promising contenders for future uses in harsh conditions.
Overall, the selection of high-temperature material depends on the specific requirements of the application, balancing factors like cost, performance, and environmental conditions. As industries continue to push the boundaries of what these substances can achieve, the development and optimization of high-performance mixtures remain a critical focus.
Applications of High Temperature Alloys
High-performance materials, characterized by their ability to withstand extreme conditions, are indispensable across various industries. In aerospace, these metals are essential for turbine engines, where the need for materials that retain strength and reliability at high temperatures is crucial. For instance, multi-principal element materials (MPEAs) have been demonstrated to provide superior performance due to their high strength, hardness, and toughness over a wide range of temperatures.
In the automotive sector, high-performance metals are used in exhaust systems and turbochargers, which must endure high temperatures and pressures. These mixtures prevent failure under extreme conditions that traditional materials like steel and aluminum cannot withstand. For instance, at temperatures near 2,500 degrees Fahrenheit, steel liquefies, and aluminum deteriorates in the presence of moisture and oxygen, emphasizing the need for stronger substances in automotive applications.
Power generation facilities employ high-performance metals in parts like gas turbines and heat exchangers. These materials must maintain their integrity and efficiency under continuous high-temperature operations. The exceptional thermal stability and corrosion resistance of these materials make them ideal for the demanding environments of power generation.
The oil and gas sector also depends significantly on advanced materials for drilling tools and valves, which face severe conditions like high pressures and corrosive surroundings. The use of Peas in these fields ensures durability and reliability, reducing downtime and maintenance costs.
‘The progress in material technology, led by research institutions such as the Johns Hopkins Applied Physics Laboratory, keeps expanding the limits of what these substances can accomplish.’. Through the creation of intricate microstructures and improving their characteristics, these materials are becoming more essential in facilitating industrial uses that require high performance in severe conditions.
Factors to Consider When Selecting High Temperature Alloys
Choosing high-performance materials for industrial uses necessitates thoughtful evaluation of several important factors. The operating temperature range is crucial, as materials must maintain their integrity under extreme heat. For example, traditional mixtures such as steel liquefy at approximately 2,500 degrees Fahrenheit, rendering them inappropriate for specific uses. Mechanical stress conditions and environmental exposure also play significant roles, as illustrated by the corrosion issues in steel compressor cases that lead to fatigue failures in blades.
Specific application requirements, such as weight limitations and thermal conductivity, are vital in determining the suitable metal mixture. For example, multi-principal element alloys (MPEAs) are gaining attention due to their high strength, hardness, and toughness over a range of temperatures, making them ideal for aerospace and nuclear power industries. Economic factors, including resource cost and availability, further affect the decision-making process. Researchers from the Johns Hopkins Applied Physics Laboratory are accelerating MPEA design by creating complex microstructures, which can provide valuable data from just a few samples.
Comprehensive testing and analysis are essential to ensure optimal performance. This includes assessing the material’s resistance to creep, corrosion, and other forms of degradation. Innovative solutions, such as the predictive tool for high-entropy materials, can forecast how new substances will behave under high-temperature oxidative environments, thus assisting in the selection process. Overall, the development and selection of high-performance materials must be meticulously managed to meet the demanding requirements of modern industrial applications.
Trends and Future Developments in High Temperature Alloys
The area of high-temperature compounds is experiencing a notable change, propelled by the need for substances that can endure extreme conditions like outer space and the Arctic. Conventional metal mixtures such as steel and aluminum struggle under extreme temperatures, cold, and pressure, resulting in the creation of sophisticated multi-principal element materials (MPEAs). These innovative substances, composed of several elements in equal proportions, exhibit exceptional strength, hardness, and toughness across a wide temperature range, making them ideal for high-stress applications.
Emerging technologies like additive manufacturing are revolutionizing the production of these materials, enabling the creation of complex geometries previously unattainable. This advancement is especially vital for sectors like aerospace and nuclear power, where substances must withstand extreme conditions. The unique characteristics of refractory high-entropy materials (Rheas), for instance, enable them to stay stable and durable under the extreme heat of jet engines or the radiation within nuclear reactors.
The global metal 3D printing market is expanding, with a focus on metals like titanium, nickel, and aluminum, segmented by forms such as powder and filament. This growth is supported by substantial investments in research and development, leading to new product launches that push the boundaries of what these advanced materials can achieve. By gathering scientists and engineers from industry, government, and academia, collaborative efforts are promoting the widespread use of these high-performance materials.
As industries strive for greater efficiency and sustainability, the demand for advanced high-temperature materials is set to increase, driving further innovations in this critical sector. The development of new alloys not only enhances performance characteristics but also opens up new applications, ensuring that these materials play a pivotal role in the future of industrial advancements.
Conclusion
High-temperature alloys represent a crucial element in modern industrial applications, demonstrating exceptional performance in extreme environments where traditional materials fail. Their unique properties, including outstanding strength, oxidation resistance, and fatigue resistance, make them indispensable across sectors such as aerospace, automotive, power generation, and oil and gas. The versatility of these alloys, particularly multi-principal element alloys (MPEAs), showcases their capability to withstand high thermal and mechanical stresses, ensuring reliability in demanding conditions.
The selection of the appropriate high-temperature alloy involves a strategic assessment of various factors, including operating temperature, mechanical stress, and economic considerations. Understanding the specific requirements of each application is vital to achieving optimal performance. As industries strive for innovation, advancements in predictive tools and manufacturing technologies are accelerating the development of next-generation alloys, enhancing their properties and expanding their potential applications.
Looking ahead, the evolution of high-temperature alloys is poised to continue, driven by the increasing demands for materials capable of withstanding extreme conditions. The integration of new technologies, such as additive manufacturing, is set to revolutionize the production processes, enabling the creation of complex geometries and enhancing performance characteristics. As the industry evolves, high-temperature alloys will undoubtedly play a pivotal role in shaping the future of material science and engineering.